Subtotal: $
Checkout-
Firewood
-
Insights on Creation
-
Conservation Is for Conservatives
-
Where Rivers Meet the Sea
-
The Psalmic Soundtrack of John Muir
-
John Muir’s Gloria in Excelsis
-
Nature and Revelation
-
Digging Deeper: Issue 4
-
The White Lily
-
Hitler’s Nemesis
-
Growing into Manhood
-
Why We Need Stories Like Homage to a Broken Man
-
Editors’ Picks Issue 4
-
Meeting Mary
-
Johnny Appleseed
-
Readers Respond: Spring 2015
-
Report from Rome
-
Together On Marriage
-
A Spark Bird Lights a Fuse
-
Can Anything Good Come from Climate Change?
-
Jesus Is Coming – Plant a Tree!
-
Our Garden, God’s Garden
-
Becoming a Rooted Church
-
A Gospel of the Ground

The maize plants growing in my experimental greenhouse were starting to flower. When I started shaking the pollen from the tassels onto the silks of individual plants, a task I’d done countless times during my years as a maize genetics researcher, something strange happened. First, I started having trouble breathing. Soon I was gasping for air and my body started swelling up; before long my eyes were swollen shut. The alarmed greenhouse manager rushed me to the emergency room. I was experiencing anaphylactic shock, an extreme allergic reaction that can be fatal without immediate treatment. As I began to recover, I broke out in a blistery rash. It took a round of strong steroids and a month before I fully recuperated.
My doctors were concerned that I had become sensitive to maize pollen, which would mean my career in maize genetics was over. When I explained to them that the maize I was pollinating was one I’d never worked with before – a new genetically engineered (GM) variety – they began to suspect the cause of my reaction. Could it be that the foreign substances in GM pollen were triggering my immune system? Tests eventually bore out their hunch.
This dramatic first brush with GM maize, which had then recently been approved for commercial sale in the United States, was almost twenty years ago. Last year, a peer-reviewed study confirmed that such allergic responses can be caused by inhalation of GM pollen.footnote In the meantime, though, my own work had taken a surprising turn – one that seems to hold the promise of an alternative to the genetic engineering not only of maize but of other major food crops as well.
A Poisoned Promise
Our ancient ancestors lived close to the earth and were keenly aware of how vulnerable their food supply was to the forces of nature. The seeds that provided their food were sacred. Grains such as maize and wheat were viewed as gifts from the gods and revered as the source of life.
Today, by contrast, most of our food is grown on large-scale industrialized farms using heavy machinery, patented seeds, and intensive use of synthetic fertilizers and pesticides. In spite of growing consumer concern, most people remain unaware of how their food is produced or the harm that industrialized farming is causing the environment and human health.
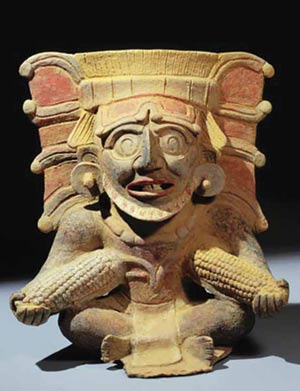
A K'iche' Maya burial urn depicting the maize god. Justin Kerr
Almost all corn, soy, cotton, canola, and sugar beets grown in the United States and Canada are genetically engineered. Such crops are known as genetically modified organisms (GMOs). Since they provide important ingredients in the production of processed foods, approximately 80 percent of all food products on grocery shelves in the United States today contain GMOs.
In order to create a GMO, researchers alter a plant by inserting a foreign gene – either a gene from another species such as a bacterium, virus, animal, or other plant, or a synthesized gene that has never occurred in nature. Researchers hitch this gene to a reporter gene so that it can enter the host plant and cause it to express the desired traits.
Most GM crops are engineered for insect resistance and herbicide tolerance (HT). Initially, HT crops were widely welcomed, because they ushered in an era of no-till agriculture. When the HT seed emerges, the field is sprayed with its associated herbicide – most often glyphosate, manufactured by Monsanto under the name RoundUp – which kills the weeds without injuring the crop. Since this procedure eliminates tilling for weed control, it promised to reduce soil erosion, carbon emissions, fuel and machinery costs, and farmers’ time and effort.
In the early years, GM crops seemed to make good on that promise: herbicide use in the United States declined. But weeds soon began to evolve resistance to the herbicides,footnote so that between 2001 and 2007 the amount of herbicide applied in the United States increased by one hundred million pounds. In parts of the country, in fact, weeds have become an even more serious problem for farmers than they were before HT crops were introduced. The industry’s response has been to engineer crops that will tolerate stronger, more toxic herbicides like 2,4-D, the active ingredient in Agent Orange.
A similar story has played out with crops engineered for insect resistance. To combat insect pests, researchers used the Bt gene from Bacillus thuringiensis, a soil bacterium; this gene acts as a plant-incorporated insecticide. Initially, Bt crops allowed farmers to spray less chemicals. But insects also rapidly evolved resistance, and farmers are now applying more insecticides to protect GMO crops than previously.footnote The unintended victims have included Monarch butterflies, whose numbers have dropped precipitously in recent years.
Such ill effects don’t seem to be outliers. Mounting evidence suggests that GM-based agriculture has decreased soil fertility, caused soil acidification through salt build-up from heavy chemical use, increased pollution of water supplies from agricultural run-off, and destroyed beneficial insects and soil microbes at an alarming rate. While there is no scientific consensus on GMO safety,footnote a number of studies have raised concerns about risks to human health.footnote The mantra of the biotechnology industry is that genetically engineered crops are necessary to produce enough food to feed the world’s burgeoning population. However, data comparing yields of GM crops to non-GM crops do not support this argument.footnote Nor do claims that it is faster to develop new varieties using genetic engineering hold up under scrutiny.footnote
In light of the harm caused by industrial agriculture and GMO technology, the United Nations has called for “a paradigm shift in agricultural development: from a ‘green revolution’ to an ‘ecological intensification’ approach.”footnote To many, such language may sound impractical and unrealistic. My research, however, suggests there are good grounds not to resign ourselves too easily to the current state of farming.
A Botanical Mystery
Agriculture first emerged around ten thousand years ago when humans began cultivating plants and living in village settlements. This shift from hunting and gathering occurred as big game was dying out when the climate warmed at the end of the last Ice Age. With climate change, species migrated into new ecological niches, bringing them into proximity with related species. This led to cross-pollinations that gave rise to hybrids with novel characteristics such as larger seeds and fruits, less shattering so seeds remained on plants during harvest, uniform ripening, and loss of hard seed coats and thorns. Modern crops originated from human selection of the genetic variation in these naturally occurring hybrids.
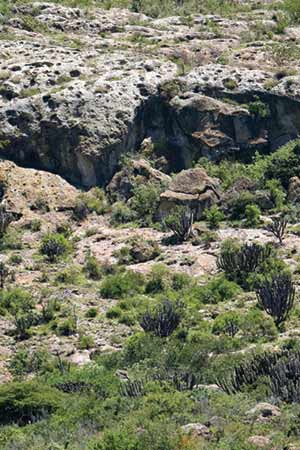
Caves in the Tlacolula valley in Oaxaca, Mexico, where the earliest evidence of domesticated teosinte, an ancestor of maize, was found. Teosinte kernels found in one cave, Guilá Naquitz, date to before 5400 BC.
Against this background, the origin of maize in particular has been one of the great botanical mysteries. The large edible ears of maize that we enjoy today – firm cobs each holding hundreds of kernels – are in fact an ancient human invention, and maize would quickly go extinct if humans did not plant, harvest, and preserve it. Yet maize does not look at all like either of its two wild relatives, teosinte and gamagrass. How did this remarkable plant arise in the first place?
That’s the question with which I began my career as an anthropologist specializing in American archaeology; my PhD research was on ancient maize. In the late 1970s, a new teosinte was discovered in the mountains of Jalisco, Mexico. This plant, perennial and more primitive than other teosinte species, sparked a new theory and new experimental research on maize’s origins.
As an archaeologist, I knew if I was to ever understand the maize story I needed to learn more about genetics. I began assisting Paul C. Mangelsdorf, a botanist famous for his theory on the origin of maize, in his new research into the origin of annual teosintes. In the process, we mapped the gene in maize coding for perennialism, the trait allowing the plant to live for two years or more.footnote This unprecedented discovery opened up a new line of research – imagine a yet-to-be-developed maize variety that didn’t need to be seeded every year, but just kept coming up every spring like many wild grasses do. I left my university job teaching anthropology to become a postdoctoral fellow in maize genetics.
Hybrid Power
In my experimental research, I made the first successful crosses between teosinte and gamagrass. The result was fertile plants that produced tiny ears resembling the oldest archaeological maize remains found in dry caves in southern Mexico.footnote Since the scientific name for Eastern gamagrass is Tripsacum dactyloides, we dubbed the resulting hybrids Tripsacorn. This discovery has profound implications for maize improvement because these hybrids are cross-fertile with maize.
Cross-fertility matters because the Tripsacorn provides a genetic bridge that breaks the sterility barrier between maize and gamagrass. Wild relatives like gamagrass are a rich reservoir of genes that enable survival under harsh conditions, so tapping their genetic diversity has traditionally been an important tool in plant breeding. In the case of maize, the rich genetic diversity of gamagrass can now be tapped to breed new strains of maize with traits for drought and heat tolerance, resistance to insects and disease, high protein, and enhanced nitrogen efficiency, reducing the need for fertilizer. In other words, this discovery opened up the possibility for sustainable maize farming.
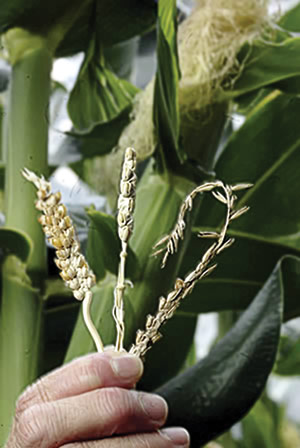
Is this what the earliest maize looked like? Ears of gammagrass, left, teosinte, right, and the gammagrass–teosinte hybrid, center, developed by the author. Image courtesy of Mary Eubanks
The first proof of concept came when I selected lines that had native resistance to corn rootworm, one of the billion-dollar bugs of maize.footnote Corn rootworm refers to beetle larvae that hatch in the soil at the time maize seedlings are sprouting and feed on their roots. Growers can lose as much as half their crop to corn rootworm damage. Accordingly, insecticides used to control corn rootworm are some of the most toxic in the world. While there is a GM variety of maize with a plant-incorporated insecticide, the insect has rapidly evolved resistance to it.
Because gamagrass is native to the plains of North America, where it co-evolved with corn rootworm, it naturally tolerates this expensive pest. In my breeding program, I selected gamagrass-introgressed maize lines with strong rootworm tolerance. Native tolerance, rather than poisoning the insects, derives its effect from air passages present in the roots, a characteristic of gamagrass. Thus, in contrast with the solid roots of maize, roots of native-trait maize do not provide adequate nutrition for the corn rootworm larvae to thrive. An added benefit of this mechanism is that the air passages enable the roots to penetrate deep into the soil below the hardpan, where they can access water. In fact, feeding by the larvae stimulates root growth, and the larger root systems boost yield and help protect against drought. In addition, the air passages attract nitrogen-fixing bacteria to the roots, reducing the need for synthetic fertilizer.
All in all, that is a fairly impressive list of beneficial traits. It’s also a revealing illustration of how we can tap ancestral genes to adapt our modern crops for ecologically sustainable agriculture. Tripsacorn maize does not destroy the insect that provides food for birds. It does not require chemical additives that kill beneficial soil organisms and cause soil acidification and compaction. Instead, it helps build up soil fertility by attracting nitrogen-fixing bacteria and improves soil permeability by naturally tilling the soil with its deep roots.
In order to test whether the native-trait lines were as good as we hoped, we conducted multi-year field trials comparing rootworm efficacy for Tripsacorn maize and Bt maize. The Bt maize had lower scores for larval feeding on the roots than the new maize; meanwhile, the new maize had bigger root systems and higher grain yields than the Bt maize. Since corn is a multi-billion dollar industry, we felt these findings were of more than just academic interest.
We submitted our results to a scientific journal for publication. The editor showed the manuscript to Monsanto, the only company at the time that had GM maize seed on the market; in response, the company threatened to sue the journal if it accepted the paper. In the end, our study was published only after we expunged the results for Bt maize. Our experience doesn’t seem to be an isolated episode; other scientists trying to conduct independent research on GM crops have reported similar treatment.footnote
Such strong-arm techniques only mask a growing problem in American agriculture. In the United States, as acreage planted in GMO crops has increased to more than 90 percent, there has been a huge shift in resources and funding into biotechnology and away from plant breeding.footnote A few companies still sell non-GM seed, but it is getting harder for farmers to find. When the price of maize dropped in 2014 to pre-biotechnology prices, however, many farmers began re-thinking the economics of planting GM crops, and seed companies are scrambling to meet a sudden demand for non-GM seed.footnote
Several US companies have made offers to license my genetic-bridge technology with the stipulation that they be allowed to convert it to GM maize. Since I want my discovery to help reduce the use of harmful chemicals, I’ve turned them down. (Tripsacorn maize is under development for the commercial market in Europe, where most countries do not plant GM maize.)
New Maize in Africa
For the foreseeable future, then, plant breeding using ancestral genes will continue to face resistance in developed countries dominated by the biotechnology-chemical treadmill. Thankfully, however, a far larger group of farmers stands to benefit much more quickly: subsistence growers in developing countries, who lack the resources and infrastructure for industrial agriculture.
In much of the world, basic crops like maize have a life-and-death importance that Westerners often fail to recognize. Although industrialized countries use maize mainly for livestock feed or as a raw material for manufactured products, it is the staple food for half the population of sub-Saharan Africa. Higher yielding than any other grain, maize is easy to digest and serves as a crucial source of carbohydrates, protein, iron, vitamin B, and minerals. It is consumed in a wide variety of forms: fresh, dried, roasted; ground and eaten in porridges, grits, and pastes; and brewed into beer. It can be stored for long periods of time and thus fills the hunger gap during the long dry season. Every part of the plant can be used to produce a variety of food and non-food products.
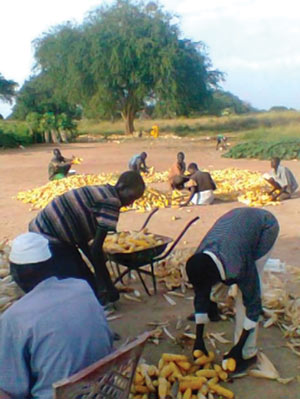
This South Sudanese school depends on produce from the school garden to help feed the children. Image courtesy of Mary Eubanks
I made the first crosses between gamagrass and teosinte in 1984, a year with a devastating drought in Ethiopia. The pictures of starving children broke my heart, and I prayed that one day my research might help relieve hunger in Africa. In 1998, a student brought me seeds of a traditional African open-pollinated maize variety he purchased in a Ghana market. I grew the tropical maize, cross-pollinated it with Tripsacorn lines, and saved the seed.
Ten years later I met Daniel Deng Bul Yak, now the archbishop of the Episcopal Church of Sudan and South Sudan. When I described this maize to him, he wanted to try growing it, and I gave him seed to take back to Sudan. It was planted in the garden of Renk Theological College in Upper Nile State, an area where the rich soil was stripped bare of all vegetation in the bitter fighting that raged in that highly contested region. Today, this maize is providing food for students in South Sudan, while seeds are being saved from each crop cycle and plans are underway to increase production to help feed refugees returning from the north and Ethiopia.
As one of Africa’s most hunger-threatened nations, South Sudan is precisely the kind of country in which ancestral-gene breeding can make the biggest impact. Millions were killed and displaced here during five decades of civil war, and the basic skills of survival in an agrarian economy were lost to a generation that grew up in refugee camps. Now that many have come home, the four-year-old Republic of South Sudan faces a massive challenge feeding its people. War ravaged the cattle herds and crops in countless villages, destroying seed and root stocks along with markets and other forms of traditional infrastructure. Chronic malnutrition is widespread.
Here the high protein content of Tripsacorn maize can be lifesaving: at 17.4 percent, it is more than double that of either sorghum (7.9 percent) or standard maize (7.8 percent). This variety also has elevated levels of essential amino acids that the body needs to build proteins it cannot produce itself.
Apart from war, another threat to food security in South Sudan and East Africa is post-harvest loss of grain: weevils consume as much as 25 percent of maize stored for the dry season. Experiments conducted by James Throne, a USDA entomologist, demonstrated that several lines of Tripsacorn maize have a staggering 100 percent native resistance to grain weevil.footnote This could be decisive for smallholder farm families whose maize is stored for months outdoors in granaries made from natural materials, or hanging from the ceilings of their homes. Other traits that can be particularly beneficial for South Sudan include resistance to insects, disease, and the African parasitic weed Striga, as well as enhanced nitrogen efficiency, enabling plant growth in poor soils.
Ancient Genes and Climate Change
Apart from poverty and war, South Sudan faces another threat, one it shares with many developing countries: global warming. Climate-change models predict increased heat and drought for much of South Sudan. Fortunately, Tripsacorn maize stands out for its very high level of drought and heat tolerance.
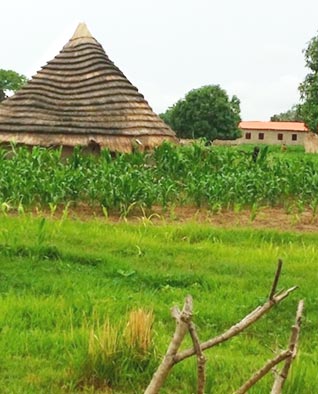
The first planting of Tripsacorn maize outside a school in Unity State, South Sudan. Image courtesy of Mary Eubanks
I had seen indications of this property of the new maize in early field observation and growth-chamber experiments. But it was not until 2011, while on sabbatical in Texas during the worst drought on record there, that I discovered just how exceptional Tripsacorn really is. I had planted a small dry-land plot at the University of Texas in Austin. Already at planting time there was a twenty-seven-inch deficit in rainfall. In the weeks that followed, no rain came. I had not installed an irrigation system, though I occasionally hand-watered when the plants appeared stressed. By early June, all the commercial-variety control plants were dead. Yet the Tripsacorn plants remained green and were producing ears. I looked forward to being able to report glowing results. Then one night raccoons came in and destroyed the entire crop, and I abandoned the plot.
When I returned in late July, at the far back of the plot I found, much to my surprise, some green maize plants. Even more surprising, they were producing silks and pollen under conditions of extreme heat and moisture deficit: the average high temperature since planting had been 37.1 degrees Celsius (98.8 degrees Fahrenheit). Although maize pollen and silks are not viable above 32 degrees Celsius, I pollinated these exceptional ears anyway. I watered them on the day of pollination, and again one week later. The temperature ranged from 39 to 40.6 degrees Celsius the days I pollinated, and the average high temperature of 40 degrees Celsius (104 degrees Fahrenheit) continued throughout the month of August. No rain fell in July or August. Even so, the maize plants bore seed, and three of the ears were completely filled out. Such extreme drought and heat tolerance in maize is unprecedented.
Ears of Tripsacorn hang outside Renk Theological College, South Sudan. Image courtesy of Mary Eubanks
I realized a seed like this could be game-changing for the millions of subsistence farmers most threatened by climate change. The following year, I worked with Nyuol Tong, a South Sudanese student at Duke University who had founded a school in his home village to help educate his people. We made crosses of gamagrass-introgressed African maize with the exceptionally drought- and heat-tolerant maize discovered during the Texas drought. In 2013 Mr. Tong took seed of several varieties we created to his home in upper Unity State, South Sudan, and it was planted in the school garden.
In this part of Africa, a school garden is no mere side project. If the school can provide a meal, children will come; for many children it may be the only food they have to eat for the day. To our joy, the first planting of the maize grew well and yielded a bountiful harvest.
Looking to the future, our goal is to select varieties well adapted to the region’s growing conditions and local food preferences. Any surplus seed left after feeding the children will be distributed to neighboring farmers and sold at market. This will generate income for the school and food security for the whole community. A long-term goal is to launch a participatory breeding program, in which the farmers learn how to grow higher yielding, locally adapted hybrid maize. The new hybrid maize could be the basis for forming an indigenous start-up seed company. In 2015, we will begin testing Tripsacorn maize in a new project in West Africa.
Working with Nature
What wider lessons can we learn from the success of ancestral-gene breeding in maize? It’s time to take an anthropological approach to agriculture, one that takes its clues from antiquity and from traditional farming practices and is oriented to the needs and preferences of local communities. By blending innovative modern science with indigenous knowledge, we can significantly enhance the food security, nutrition, and health of resource-poor farmers without undermining their cultural heritage or damaging the environment.
Improving crops through hybridization with wild relatives is a time-honored tradition in plant breeding. The model presented here is a promising alternative to the increasing use of GMOs and agrichemicals in Africa and elsewhere. It’s an approach that works with nature, introducing traits that evolved naturally and thus do not lose efficacy over time. This is one way we can fulfill the United Nations’ mandate to move away from the “green revolution” based on genetically modified seeds and heavy use of fertilizers and pesticides to an approach that is sustainable and regenerative, enabling farmers to be good stewards of the earth.
Footnotes
- M. Andreassen, E. Rocca, O.G. Wikmark, J. Van der Berg, T. Traavik, “Humoral and cellular immune response in mice after airway administration of Bacillus thuriengensis Cry1Ab and MON810 cry1Ab-transgenic maize,” Food and Agricultural Immunology (2014).
- Major M. Goodman, James B. Holland, and Jesus Sánchez G., “Breeding and Genetic Diversity,” in Genetics, Genomics and Breeding of Maize, ed. Ramakrishna Wusirika, Martin Bohn, Jinsheng Lai, Chittaranjan Kole (Boca Raton, FL: CRC Press, 2014), 14–50.
- Aaron J. Gassman, Jennifer L. Petzold-Maxwell, Ryan S. Keweshan, Mike W. Dunbar, “Field-evolved Resistance to Bt Maize by Western Corn Rootworm,” PLOS ONE 6, no. 7, (2011).
- European Network of Scientists for Social and Environmental Responsibility, “No Scientific Consensus on GMO Safety,” ENSSER Statement, 21 October 2013.
- Mary Eubanks, “Allergies á la Carte: Is There a Problem with Genetically Modified Foods?” Environmental Health Perspectives (March 2002): A130.
- Jorge Fernandez-Cornejo, Seth Wechsler, Mike Livingston, and Lorraine Mitchell, “Genetically Engineered Crops in the United States,” ERR Report 162, United States Department of Agriculture Economic Research Service (2014): 12.
- Major M. Goodman, “New Sources of Germplasm: Lines, Transgenes, and Breeders,” in Memoria Congresso Nacional de Fitogenetica, ed. J.M. Martinez, F. Rincon S., and G. Martinez G. (Saltillo, Mexico: Univ. Autonino Agr. Antonio Narro, 2002), 28–41.
- United Nations Conference on Trade and Development, “Wake Up Before It’s Too Late: Make Agriculture Truly Sustainable Now for Food Security in a Changing Climate,” UNCTAD Trade and Environment Review 2013.
- Paul C. Mangelsdorf and Mary Eubanks Dunn, “Linkage relations of Pe*-d,” Maize Genetics Cooperation Newsletter 58: 53.
- Mary Eubanks,“A Genetic Bridge to Utilize Tripsacum Germplasm in Maize Improvement,” Maydica 51 (2006): 315.
- D. Prischmann, M. Eubanks, K. Dashiel, D. Schneider, “Evaluating Tripsacum-introgressed Maize Germplasm After Infestation with Western Corn Rootworm (Coleoptera: Chrysomelidae),” Journal of Economic Entomology 133 (2009): 10.
- Emily Waltz, “Under Wraps,” Nature Biotechnology 27, no. 10, (October 2009): 880. See also Emily Waltz, “Battlefield,” Nature 461, (3 September 2009): 27.
- Bill Tracey and Michael Sligh (eds.), Proceedings of the 2014 Summit on Seeds and Breeds for 21st Century Agriculture (Pittsboro, North Carolina: Rural Advancement Foundation International, RAFI-USA, 2014).
- Jacob Bunge, “Fields of Gold: GMO-Free Crops Prove Lucrative for Farmers,” Wall Street Journal, February 2, 2015.
- James E. Throne and Mary Eubanks, “Resistance of Tripsacorn to Storage Insect Pests,” Journal of Stored Products Research 28 (2002): 239.
Mary Eubanks is an adjunct professor of biology at Duke University and founder and president of Sun Dance Genetics, a company that is developing drought- and disease-resistant corn varieties. In collaboration with African partners, she works to make seeds from these new varieties available to small farmers in South Sudan and West Africa.
Already a subscriber? Sign in
Try 3 months of unlimited access. Start your FREE TRIAL today. Cancel anytime.